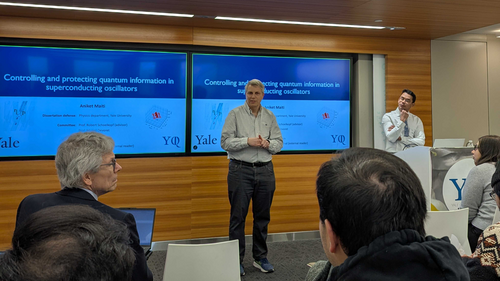
On March 4, 2025, Aniket Maiti successfully defended the thesis “Controlling and protecting quantum information in superconducting oscillators” (advisor: Robert Schoelkopf).
Maiti explained, “We’re entering a new era of extremely well-controlled experiments where we directly manipulate the quantum states of complicated systems for potentially exponential gains in sensing and computation. My work at Yale focused on a particularly interesting form of quantum information, that stored in the continuous variable phase-space of microwave light. The systems I built allowed the control of microwave light in multiple oscillators down to a single photon (that’s less than three-millionths of an attojoule of energy) with ultra-high fidelity. This gave rise to a new quantum processor architecture and many interesting investigations into the interaction of light and matter!”
He will be working as a Research Scientist at Google Quantum, helping them build scalable fault-tolerant quantum computers.
Thesis abstract
Modern quantum experiments allow the precise manipulation and measurement of many-body quantum states, pushing quantum mechanics from a testable theory to a utilizable technology. The central promise of these systems is to process quantum information for exponential advantages in computing, sensing, and communication. An interesting way to achieve such a processor is to store and manipulate quantum information in the continuous-variable (bosonic) phase space of light. Since photons in free-space do not talk to each other, such an approach necessarily requires the introduction of nonlinearity through strong light-matter couplings. However, since all matter is lossy, this inevitably introduces a trade-off between the speed of control and the inherited decoherence of the light.
This thesis achieves controllable bosonic systems by trapping microwave light in the standing waves of a superconducting oscillator, and making it interact with Josephson junction-based nonlinearities. I first demonstrate novel ways to exchange single photons between two oscillators through carefully constructed driven nonlinearities, achieving several orders of magnitude higher fidelity than previously possible. I then introduce ways to utilize such a high-fidelity coupler to dynamically couple light and matter, in a way that breaks the trade-off between universal control and inherited decoherence. Finally, I theoretically show how such universal control can autonomously protect any appropriate error-correction code in the bosonic mode, against a full Lindbladian error channel. Together, this thesis provides a promising path towards fault-tolerant bosonic quantum processors.